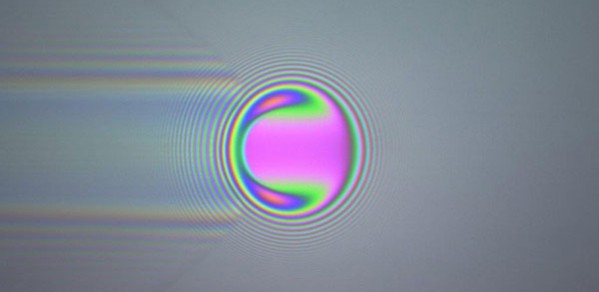
The 9th of March 2016 is the 50th anniversary of the publication of the report (now known as the Jost report) which first coined the word 'tribology' (the science and technology of friction, wear and lubrication) and stimulated research and innovation in this pervasive enabling technology.
The Cambridge connection to the subject dates back to the very important work of Philip Bowden and David Tabor from the 1940s to the 1980s (though Bowden died in 1968), initially as part of the Dept of Physical Chemistry, then in the Cavendish. The history of their group can be found in a link to the right.
Within the Department of Engineering really important work was done by Ken Johnson (who died last year) and Jim Greenwood (Emeritus Reader).
Professor Ian Hutchings has written an article for the magazine Ingenia which follows below.
The control of friction and wear in mechanical systems by lubrication and surface engineering has led to safer, faster transport as well as medical innovations. Ian Hutchings FREng, GKN Professor of Manufacturing Engineering at the University of Cambridge, highlights the progress and some failures of the important discipline of tribology.
Although the study of friction, lubrication and wear in machines dates back at least as far as Leonardo da Vinci’s remarkable contributions in the 15th century, it is only 50 years since a single word, ‘tribology’, was coined to describe this broad field of knowledge. Even now, half a century later, that term is still not widely known, nor is it generally recognised as a key enabling technology.
WHAT IS TRIBOLOGY?
In 1964, a working group chaired by Dr H Peter Jost CBE, was invited by the UK Department of Education and Science to investigate the state of lubrication education and research, and to give an opinion on the needs of Industry.
The birth of the term ‘tribology’ is linked to this report on lubrication delivered to government by Dr H Peter Jost CBE in 1966
When the group reported in February 1966, it proposed a new word to describe this multidisciplinary field – tribology, from the Greek root τριβο meaning rubbing or attrition. The Oxford English Dictionary defines tribology as “the branch of science and technology concerned with interacting surfaces in relative motion and with associated matters (as friction, wear, lubrication, and the design of bearings).”
Many would also include the field of surface engineering – modifying or coating a surface in order, for example, to achieve lower friction or greater wear resistance. The interaction of surfaces is complex. One of the pioneers of quantum physics, Wolfgang Pauli, famously said: “God made the bulk, surfaces were invented by the devil”.
Progress in tribology has demanded the skills of mechanical engineers, materials scientists, physicists and chemists. And in turn, advances in tribology have underpinned much of the world’s engineering progress. Tribology is most often associated with bearing design, but its reach extends to all aspects of modern technology in which surfaces move against each other, even to apparently surprising areas such as hair conditioners and cosmetics.
Any mechanical system where one material slides or rubs over another will be affected by complex tribological interactions, whether lubricated like engine bearings, hip implants and other artificial joints, or unlubricated, as in metals sliding at high temperatures. In these conditions, conventional lubricants cannot be used, but the formation of compacted oxide glaze layers can protect against wear.
BEARING PROGRESS
Any mechanical system where one material slides or rubs over another will be affected by complex tribological interactions, whether lubricated like engine bearings, hip implants and other artificial joints, or unlubricated, as in metals sliding at high temperatures. In these conditions, conventional lubricants cannot be used, but the formation of compacted oxide glaze layers can protect against wear.
A hard disk head and arm on a platter. The read-write head at the end of a movable supporting arm ‘flies’ over the surface of the rotating magnetic disk at a height of less than 10 nanometres, supported on a film of air that acts as a lubricant. Hard disk drives typically contain a stack of several disks, with heads on both surfaces of each disk © Eric Gaba, Wikimedia Commons user Sting
Bearings are key components in nearly all mechanical systems – they transmit forces while imposing minimal frictional drag – and are very often taken for granted. Sometimes the load is carried by rolling elements – steel balls or rollers – which run against precisely profiled raceways, and even these apparently simple components have seen remarkable progress in the past half-century.
Until relatively recently, the life of rolling bearings was limited by fatigue cracking. Cracks initiated and grew from microscopic defects under the surface of the steel, eventually reaching the surface where they led to detachment of material, the formation of a rough, pitted profile and unacceptable vibration, or even to gross fracture of the whole bearing structure. Rolling contact fatigue is now almost unknown in modern bearings, thanks to our understanding of the process and to improvements in steelmaking and refining which have virtually eliminated the damaging oxide and sulfide inclusions from which the fatigue cracks initiate.
Another type of bearing, the gas bearing, in which the load is carried by a very thin aerodynamically-generated film of (usually) air, is a key component in the computer hard disk drive. The data storage capacity of these devices has steadily increased, from the IBM 1302 in 1963, which was the size of a small car and stored 120 megabytes at a cost (in 2016 terms) of almost 2 million dollars, to the palm-sized drive of the present day, storing perhaps four terabytes for around 100 dollars. This massive increase in capacity has been achieved by a progressive reduction in the size of the magnetic domains in which the data is stored, as well as by advances in the physics of the read-write process, but it has also involved a remarkable reduction in the ‘flying height’ of the head over the disk surface – and this last advance can be credited to tribology.
IBM’s drives of the 1960s were the first to support the read-write heads on thin aerodynamic films, about 6 micrometres thick. Now, in modern drives, the heads fly at less than 10 nm, a feat that demands not only precise modelling of the air film and head dynamics but also the production of disk surfaces of extraordinary flatness, protected by a 15 nm coating of hard carbon and a lubricant layer only one nm (about 10 atoms) thick.
While modern computing power undoubtedly benefits from tribology, some key advances in tribology have, in turn, been greatly assisted by developments in computing – see Elastohydrodynamic lubrication.
ELASTOHYDRODYNAMIC LUBRICATION
In some bearings, such as the crankshaft journal bearings in a car engine, the load is carried by a hydrodynamically-developed oil film. Here the pressure is quite low and elastic deformation of the surfaces can be ignored, at least to a first approximation. But where the contact stress is high, as in the contact between gear teeth, cams and followers, or in ball or roller bearings, the simple hydrodynamic theory is useless – we must take account not only of the elastic distortion of the surfaces, but also of the fact that the viscosity of the oil rises very rapidly with pressure.
Gear teeth make contact over a small area, resulting in a very high local pressure as shown in this finite-element model. This distorts the surfaces of the teeth, and also compresses the oil between the teeth so that its viscosity momentarily becomes very high. The same phenomena occur in ball and roller bearings, and the lubrication conditions are termedelastohydrodynamic © Dr Rhys Jones, University of Warwick
In the tiny gap between two gear teeth, the oil behaves momentarily like an extremely viscous liquid, preventing metallic contact and allowing the gears to run with minimal wear.
The local power density is remarkable – of the order of 100 TW/m3, equivalent to dissipating the entire electrical power output of the UK in the volume of a can of fizzy drink. Modelling these elastohydrodynamic phenomena, by coupling the fluid mechanics of the lubricant film with the elastic distortion of the gap and the effect of pressure on the oil, is complicated and computationally demanding.
The first such calculations were made for the simplest line-contact configuration in the late 1950s, but it was to be another two decades before elliptical contacts could be handled. Modern computational models can now accurately predict the performance of contacts involving rough and patterned surfaces, fluids with complex rheology, and thermal effects. Modelling the commonly-encountered regime of ‘mixed’ lubrication, in which the lubricant film only partially separates the surfaces, and is so thin where it is present that continuum models may be invalid, remains challenging.
HEALTHCARE ADVANCES
Hip replacement provides perhaps the most striking example of a tribological advance in medicine. It is now a routine procedure, and about 100,000 patients receive a total hip replacement (THR) each year in the UK. Worldwide, 10% of men and 18% of women over the age of 60 have symptomatic osteoarthritis, which is one of the ten most common disabling diseases in developed countries.
Modern total hip replacements use a very smooth ball (metal or ceramic) attached to the top of the femur. The ball slides within a spherical socket, usually made from ultra-high molecular weight polyethylene or ceramic, fitted into the pelvis © AAOS
Although the artificial hip joint existed for many years, it originally provided a primitive and unsatisfactory solution to severe osteoarthritis. In the 1960s, Sir John Charnley pioneered modern total hip arthroplasty at Wrightington Hospital, Wigan, and spent the next two decades refining the replacement joint and developing the procedures to implant it.
Modern hip prostheses have evolved considerably but most still replace the body’s natural ball-and-socket joint with a very smooth metallic (stainless steel or cobalt-chromium alloy) ball at the head of the femur, articulating in a cup in the pelvis made from ultra-high molecular weight polyethylene. Smaller, but still substantial, numbers use an alumina ceramic ball with a polyethylene cup, or ceramic for both components. The challenge to the designer is to reproduce the ease of movement and range of articulation of the natural joint, while achieving secure attachment to the bone and effective operation for as long as possible. Current artificial joints are lubricated by naturally occurring fluid in the body and the surfaces experience very low wear rates. Typically, around 4% of joints may need replacing, for various reasons, after seven years.
There was, however, a period in the early 2000s when there were problems with some artificial hip joints. A new design of THR had been introduced that had a metal femoral head articulating in a metallic (rather than polymer) cup. These joints needed to be replaced at a significantly higher rate than the metal/polyethylene, ceramic/polyethylene or ceramic/ceramic designs. The causes of the failure were mostly tribological – while in many patients the metal-on-metal bearings performed well, in some cases there was an adverse reaction to metallic debris and ions (such as cobalt and chromium from the alloy used) released into the body by wear of the joint.
Understanding the complex lubrication and wear processes which occur in the body has been vital to optimising the design of these important life-enhancing devices. The field of medical tribology now extends much more widely than hip replacements, from other types of prosthetic joints to the friction of surgical gloves and catheters, the lubrication of contact lenses, and even the cleaning and wear of teeth.
TRANSPORT TRIBOLOGY
Transport inevitably involves surfaces in relative motion, and tribological advances have made substantial contributions to travel by land, sea and air. Bearings lie at the heart of most transport systems, and sliding and rolling interfaces are critical features of most sources of motive power. The past 50 years have seen major gains in fuel efficiency in road transport and passenger aircraft – in part due to changes in the underlying design of the power plant, but also attributable to tribological improvements.
Under-platform friction dampers – small fillets of metal which fit between the separate turbine blades in the hottest part of a jet engine. Turbine blades are held by their ends in slots in the rim of a rotating disk and can vibrate like tuning forks. Friction against small wedge-shaped inserts fitted between neighbouring blades helps damp out the vibration, significantly extending the life of the blades
Frictional losses are significant in road vehicles – they account for one third of the energy generated from the fuel in a modern passenger car, with half of this being lost in the engine and transmission. These losses have been progressively reduced over time by the use of thinner oils, which cause less viscous drag in the bearings. However, lowering the oil viscosity inevitably reduces the thickness of the oil films which separate the sliding and rolling surfaces. As oil films become thinner, the moving surfaces come into ever-closer contact and chemical lubricant additives are needed to control the friction and wear which would otherwise become unacceptable.
The most effective additives contain elements such as zinc, phosphorus and sulfur, which can degrade the performance of exhaust catalysts and also have undesirable environmental impacts so that challenges in formulating new lubricant additives remain. There is scope for further reduction in frictional losses through the development of improved lubricants, the use of coatings, and by controlling surface textures in engine and drivetrain components.
There have also been improvements in aero engine performance. Since the mid-1960s, these have been spectacular – whether success is measured by turbine entry temperature, thermal efficiency, overall pressure ratio or specific fuel consumption. In addition, these improvements have been matched with increased durability. Advances in materials and aerodynamic design have been vital here, but tribology and surface engineering have also contributed significantly – not only in the numerous bearings, gears, seals, splined joints between shafts and lubricants used in modern jet engines, but also in more specialised areas.
One of these has involved the use of abradable coatings to control turbine and compressor blade tip clearances. Another is the thermal barrier coatings that now allow first-stage turbine blades with internal cooling passages to operate in a gas stream that is considerably hotter than the melting point of the blade alloy. A further example is the use of ‘under-platform friction dampers’, tiny fillets of metal which fit between the separate turbine blades in the hottest part of the engine. Microscopic sliding movement between the blade and the fillet is allowed, which damps out unwanted blade vibrations through friction, and thereby increases the fatigue life of the blades by a factor of two or more.
The machining operations needed to manufacture not only aero-engine components but also a wide diversity of products now almost universally use coated cutting tools. Unheard of 50 years ago, this advance in surface engineering has substantially reduced tool wear and friction, enabling higher production speeds and lower costs.
TRIBOLOGICAL FAILURES
Tribological phenomena are fundamentally important to the operation of so many mechanical systems. However, designers and operators have sometimes missed or overseen potential problems, and there are clear examples where failures in engineering systems have tribological origins.
Gauge corner cracking’ (cracks on the gauge corner) and ‘head checking’ (cracks on the head of the rail) are both examples of the more general phenomenon of RCF which occurs in bodies in rolling contact. Such bodies can damage one another in various ways depending upon the severity of the contact pressure and the shear forces in the area where the bodies come into contact. For most trains in Britain this ‘contact patch’ is about the size of a five pence coin, and the behaviour in this contact patch creates the forces between the wheel and rail that lead to RCF – see Why rails crack (Doherty, Clark, Care and Dembosky) Ingenia 23
A tragedy familiar to many in the UK was the Hatfield rail crash in October 2000. An Intercity express train travelling on the East Coast Main Line derailed at 185 km/h; four passengers lost their lives and more than 70 were injured. One rail was found to have fractured into more than 300 pieces over a distance of 35 m. The root cause of this catastrophic disintegration was extensive rolling contact fatigue (RCF), which cracked the rail, a progressive process that should have been foreseen and controlled by periodic grinding of the rail head.
A further example of a failure in maintenance procedures, from the aviation industry, is provided by the loss of Alaska Airlines flight 261 with 88 passengers and crew in January 2000. This McDonnell Douglas 83 aircraft crashed into the Pacific Ocean when control was lost of the horizontal stabilizer in the tail. The stabilizer position was controlled by a jackscrew, which failed due to inadequate lubrication and consequent wear of the screw and its mating nut.
Faults in components of consumer products can also be dangerous, even lethal, in their consequences and costly to rectify. There are examples where either too much, or too little, friction has caused problems. In 2010, Toyota recalled 4.1 million vehicles worldwide to modify their accelerator pedals. These pedals were designed with a ‘friction pad’ intended to modify the feel of the pedal, which under some conditions could lead to the pedal returning either slowly, or even not at all, to the engine idle position after being depressed.
More recently, in 2014, GM recalled 23 million vehicles in the US to replace ignition switches. In this case the torque needed to turn the switch away from the ‘run’ position was too low, and a nudge from the driver’s knee was occasionally enough to cause the engine to stall when the car was moving; by the same action the airbag circuit was also disconnected. Here, more friction in the mechanism would have been safer.
THE NEXT 50 YEARS
Since the 1980s, new areas of tribology have emerged for which new names have been coined. These include nanotribology, biotribology, and even ‘green’ tribology. These interdisciplinary areas study friction, wear and lubrication at the nanoscale (for example, by molecular dynamics modelling and by measuring the friction on the tiny stylus of an atomic force microscope), in biological systems (including human prosthetic joints and dental materials, and also the tribology of natural and biomimetic structures), and in the ecological aspects of friction, lubrication and wear (such as tribology in clean energy sources and environmentally-friendly lubricants).
Tribological phenomena occur at the heart of most modern engineering systems and many manufacturing processes. Inevitably the future of tribology will be interlinked with technological progress. While it is impossible to predict what the future may hold, it is certain that wherever things move there will be tribological phenomena at work, and the key tribological issues of friction and wear to solve by design, materials selection, lubrication and surface engineering – whether in transport, generation of energy, healthcare, agriculture, water supply or elsewhere.
Although our understanding of and ability to model many aspects of tribology has increased enormously over the past 50 years, bridging the gulf between what happens on the atomistic and macroscopic scales remains very challenging. While we may have identified the fundamental physical and chemical processes at work in a tribological contact, combining them and applying them in a quantitative model is remarkably hard. As Pauli suggested, fiendishly complex phenomena can occur on rubbing and sliding surfaces, involving progressive changes to the local material microstructure, topography and chemistry. Prediction of friction and wear is still only possible in some rather restricted cases, and progress in the field will only come from collaborative research between engineers and scientists from several disciplines.
What is clear is that the engineers of the future will need to be aware and informed about tribology, and that in order to avoid some of the mistakes of the past, management at all levels also needs to appreciate the importance of the application of tribological knowledge. A recent international survey by the US Society of Tribologists and Lubrication Engineers found almost two thirds of the nearly 1,000 respondents to be dissatisfied with the public’s understanding and awareness of the subject. A very fitting way to mark the 50th anniversary of the Jost report would be to ensure that the essential elements of tribological understanding lie at the core of the education of every engineer.
UK INITIATIVES
For many years, professional institutions in the UK have had their own separate but thriving tribology groups, reflecting the multidisciplinary nature of the subject. In 2015, they collaborated in a new initiative. Five key learned societies (The Institution of Engineering and Technology, Institution of Mechanical Engineers, Institute of Materials, Minerals and Mining, Institute of Physics and the Royal Society of Chemistry) have come together to form UK Tribology. This body has a largely outward-facing remit to reach as much of UK industry as possible, to encourage the uptake of best tribological practice. It also has a core mission to engage with schools, colleges and universities to ensure that the importance of tribology is appreciated by students.
BIOGRAPHY
Ian Hutchings FREng is GKN Professor of Manufacturing Engineering at the University of Cambridge. He has a background in materials engineering and is the author of a widely used textbook on tribology. He was Editor-in-Chief of the international journal Wear from 1998 to 2012, and has jointly run a short course on tribology for industry for the past 25 years.
This article was first published in Ingenia, the Royal Academy of Engineering magazine, issue 66, March 2016.
Every year since 1993 John Williams and Ian Hutchings have been running a three-day annual course for industry which attracts 30-40 delegates each year from all over the world - the next one is in September 2016: http://www.ifm.eng.cam.ac.uk/events/tribology2016/